Banner: A farmer visits an inter-cropped field at a farming community in the Dominican Republic. Photo by MJC, 2018.
Sustainable Agriculture has become a popular theme in development circles. In fact, it appears everything needs to be “sustainable” these days. However, the focus on EMI projects is the inclusion of Sustainable Agriculture where appropriate for client ministries. This is so the project – whether it be a school, hospital, or orphanage – can be maintained (or supported to the desired degree or target) by production of a reliable supply of food.
There are many definitions of “sustainability”, depending on the context and the type of project. Generally, sustainability in EMI projects includes economic, environmental and social aspects. The “sweet spot” of the Venn diagram (below) is where sustainability can be achieved (Choi, 2015).Note 1 In addition, the consequences of including two aspects but not the third is apparent.
Consider, for example, the effects of open cast mining. This may provide economic benefits for the mining company and resources for industry, as well as social benefits in the form of employment and income for the community. However, it can be deficient in the environmental aspects of scarred landscapes left behind. This may be an “Equitable” situation but not a “Sustainable” one since it involves the exploitation of a non-renewable resource.
Current thought on sustainability has covered the three topics above: Environmental, Social and Economic - sometimes with varying degrees of emphasis on each topic. When considering agriculture, the situation is somewhat different. Agriculture is an ongoing activity and is anticipated to be under continuous review. Implicit in the ongoing discussions of Sustainable Agriculture is the (usually unstated) assumption that if something is sustainable, it will last forever!
However, there is a saying:
“In the short term, everything is sustainable.
In the long term, nothing is sustainable!” (Anon)
Accordingly, there is a fourth aspect that has received little attention: This is the Time Period for which something is considered to be sustainable. An alternative way of looking at Sustainable Agriculture is to address sustainability as a process – an ongoing evaluation to ensure agricultural productivity is maintained. One of the primary measures of agricultural productivity is yield. Monitoring of yield on a continuing basis will provide an indication of changing conditions and prompt the necessity for further evaluation.
In certain contexts, sustainability has an implicit timeframe. Buildings, for example, are generally designed for an anticipated lifespan of 50 years. Dams and bridges are usually considered for longer periods due to their community importance and since their failure would have a wider impact.
Following research at Colorado State University, an investigation of agricultural practices in the western US indicated that a 50-year (or two generation) time period was appropriate for consideration of technology in current use (Sands and Podmore, 1999).Note 2 The factors influencing this choice were the anticipated time-periods for technological and sociological changes to take place. After this time, the surveyed practices were considered to have changed and were no longer valid for the analysis.
When applying this research concept in the majority world, where technological changes were anticipated to be slower and social changes slower still, a time-period of 100 years (or four generations) was estimated. This is only an estimate based on experience, however. Developments such as the adoption of mobile phone technology in majority world countries shows that the impact of technology changes needs periodic evaluation.
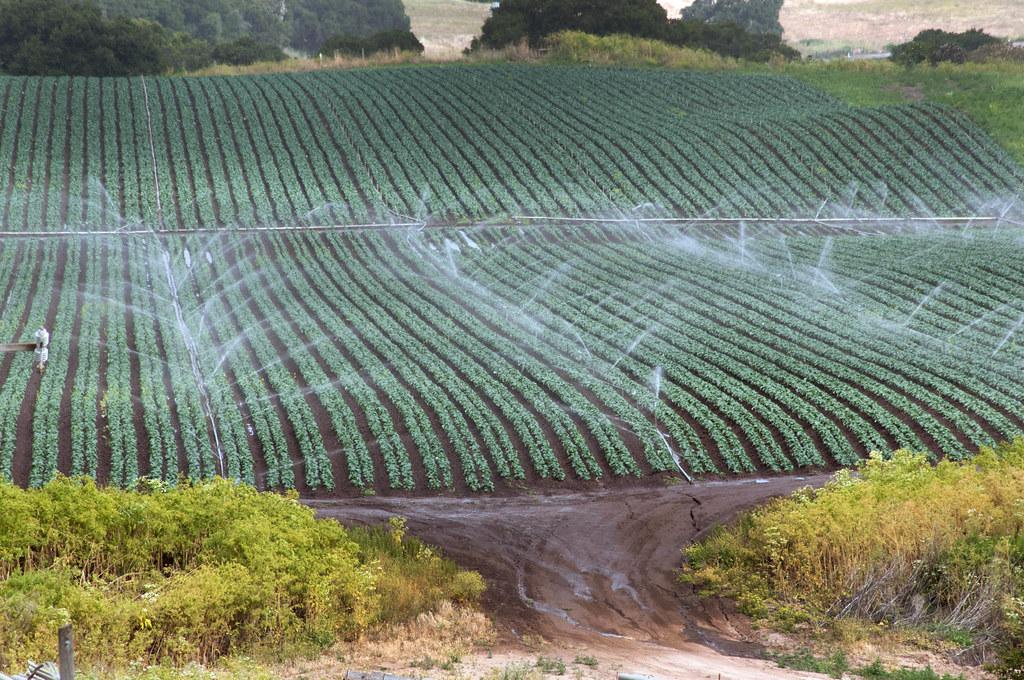
Time-period is an important factor for sustainability as technology can rapidly alter farming methods and yield. Left: A laborer manually directs irrigation water into farm furrows in the Dominican Republic (MJC, 2018). Right: A network of sprinklers irrigates a field in California, USA (USDA, photo by Lance Cheung)
Adoption of changing technologies in some areas of the majority world can be more rapid and these emerging advances may invalidate the 100-year time-period. However, in some parts of the world, settled agriculture has been going on for some 9,000 years. Farmers are generally “risk averse” and so little may have changed over this period since changes in practices might adversely affect yields. This is the case when agriculture is the livelihood and the survival of the farmer and his family from one crop season to the next is at stake.
Accordingly, the 100-year time-period is proposed as a starting point when considering agricultural technologies and practices for EMI projects. When proposing a cultivation practice or cropping system, attention must be paid to the economic, environmental, and social impacts. The impact of agricultural practices are considered within the selected timeframe. (Please note that for animal-based agriculture, the considerations are different since the useful lifespan of the animals must be included.)
However, there is little current research to suggest firm guidelines. In addition, “best practices” based on local experience need to be defined before they can be adopted. As an example, the evaluation of cropped agriculture for sustainability can be considered:
For a given farming practice, an appropriate time-period for sustainability must be established – say 50 years. While monitoring of the agricultural system may be ongoing, a thorough re-evaluation should be performed at the 25-year halfway point to ensure conditions are stable. Obviously, only those aspects that change need investigation. At one time, climate would have been considered as constant but that is no longer the case. Soil structure can change, but only slowly, while soil fertility varies more quickly. This evaluation process gives a basis for applying the criteria to the farming system on a timely basis. The object is to identify modifications to the farming system so that its productivity can be maintained.
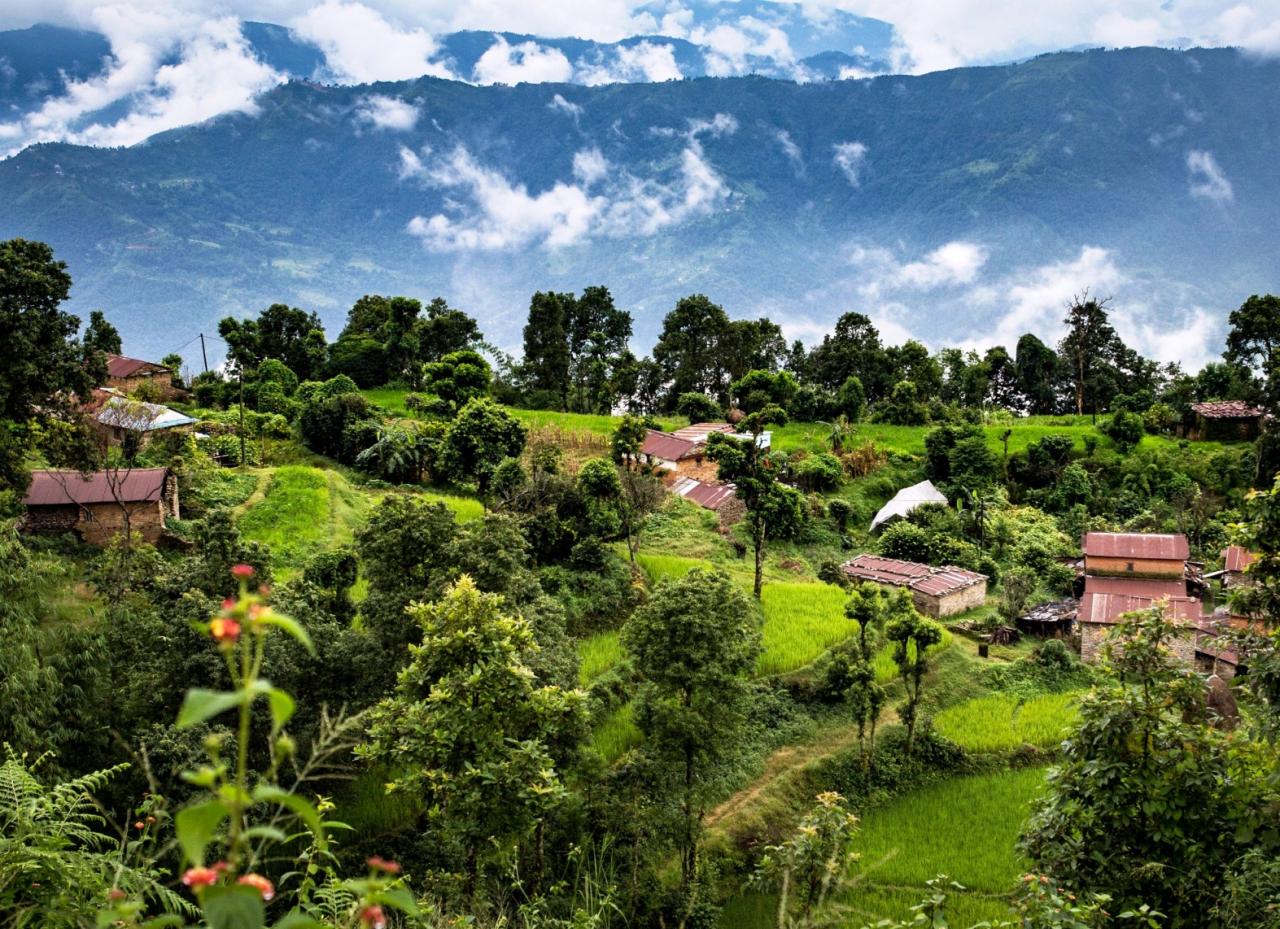
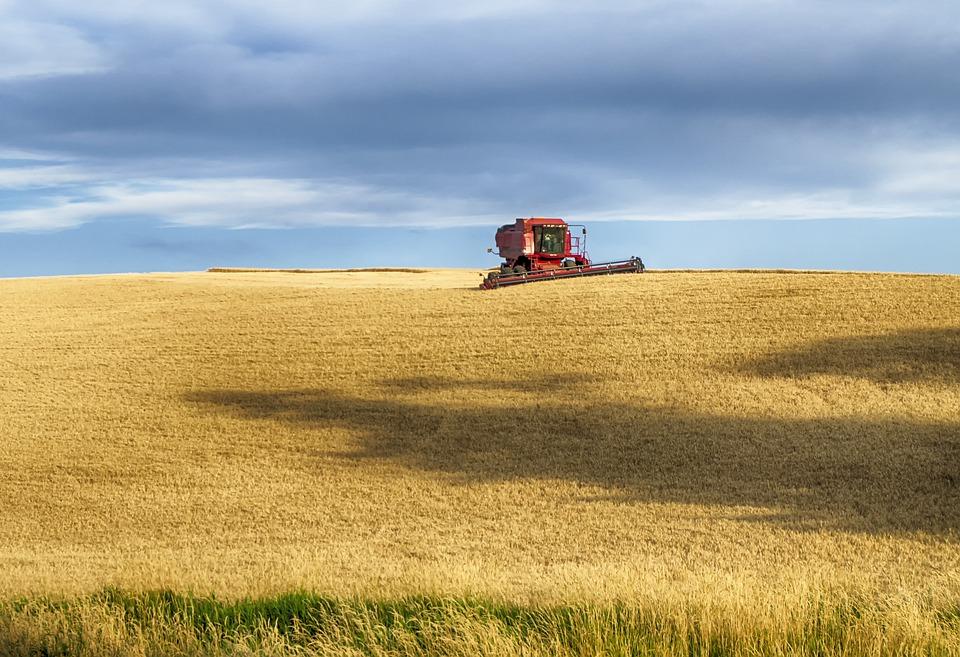
There are obvious differences between rice terrace farming in Nepal (K.Keiter, 2019) and wheat farming in Idaho, USA. To plan for sustainable agriculture and evaluate its ongoing sustainability, however, the evaluation of a host of factors is required.
Factors considered in an evaluation are given below. It should be noted that some of these categories may be predefined by the nature of the location, altitude, and climate under consideration. These conditions should be documented to recognize the opportunities for and limitations on agriculture at a given site:
- Climate – inferred from location of the site under consideration.
more definition - Topography – determined from the nature of the site, usually developed from site inspection and a topographic survey.
more definition - Soils – information obtained from the site, assisted by soils maps. (Soils maps typically available are on a large scale and only provide a general indication of the soils in the area.)
more definition - Water Supply – where water is required for crop growth and development in excess of natural rainfall in the form of irrigation.
more definition - Cropping System – the crops grown will be determined by local needs and the crop types and varieties available.
more definition - System Management – assessing the level of management present on the system.
more definition - Tillage and Harvesting Equipment – mechanization is a significant agent in the improvement of productivity.
more definition - Drainage Requirement – the removal of excess water from the site to ensure good growing conditions for the crops.
more definition - Socio-Economic Considerations – agriculture is a social as well as a technical undertaking.
more definition
While this list may appear daunting, many of the considerations can be determined by location, literature review, and/or previous experience. The identification of knowledge gaps can be valuable in determining the data to collect on a site visit or in interviews with local personnel. Site investigation becomes an important activity and adequate time must be devoted to determining the site conditions and local practices. The sources of local knowledge, such as Agricultural Extension Services and research stations also need to be identified.
More than a buzzword, sustainability related to agriculture has a particular context and depends on many variable aspects covering a wide range of technical and social considerations. Ongoing review of these factors within a selected time-period will indicate adjustments to the production system that will enable productivity to be maintained.
Choi, Y. 2015. Intermediary Propositions for Green Growth with Sustainable Governance. Sustainability 7(11), 14785-14801 (https://www.mdpi.com/2071-1050/7/11/14785, accessed March 23, 2020).
Sands, G.R. and T.H. Podmore. 1999. A generalized environmental sustainability index for agricultural systems. Agriculture, Ecosystem and Environment 79: 29-41.